C. Gillis1 and P. E. Wischmeyer2
Summary
1 Nutrition Lead, Peri-operative Program, McGill University, Montreal, QC, Canada 2 Professor of Anesthesiology and Surgery, Director of Peri-operative Research, Duke Clinical Research Institute, Duke University School of Medicine, Durham, NC, USA
Pre-operative nutrition therapy is increasingly recognised as an essential component of surgical care. The present review has been formatted using Simon Sinek’s Golden Circle approach to explain ‘why’ avoiding pre- operative malnutrition and supporting protein anabolism are important goals for the elective surgical patient, ‘how’ peri-operative malnutrition develops leading in part to a requirement for pre-operative anabolic preparation, and ‘what’ can be done to avoid pre-operative malnutrition and support anabolism for optimal recovery.
Correspondence to: C. Gillis Email: chelsia.gillis@ucalgary.ca Accepted: 11 August 2018
Keywords: nutrition prehabilitation; peri-operative nutrition; pre-surgery optimisation Twitter: @Paul_Wischmeyer
Introduction
In the peri-operative period, the primary nutrition goals are to evaluate the patient for pre-existing malnutrition, treat malnutrition to optimise surgical readiness, minimise starvation, prevent postoperative malnutrition, and support anabolism for recovery [1]. Although additional nutritional considerations will be required for surgical subspecialities and to provide personalised patient care, these basic nutrition principles hold true for all cases. Our paper uses Simon Sinek’s Golden Circle approach [2] to apply these basic surgical nutrition principles to the pre-operative period, largely focusing on elective abdominal surgery.
Surgical stress response
An understanding of the surgical stress response is essential to understanding the role nutrition plays in promoting optimal surgical recovery. Surgical trauma induces a state of metabolic activation (the surgical stress response) that parallels the extent of injury, and which is characterised by hormonal, haematological, metabolic and immunological
changes [1, 2]. The surgical stress response is clinically manifested as salt and water retention to maintain plasma volume; increased cardiac output and oxygen consumption to maintain systemic delivery of nutrient and oxygen-rich blood; and mobilisation of energy reserves (glycogen, adipose, lean body mass) to maintain energy processes, repair tissues and synthesise proteins involved in the immune response [2].
Nutritionally-relevant clinical consequences of the surgical stress response include hyperglycaemia and whole-body protein catabolism [1, 2]. Catabolism manifests clinically as the wasting of lean tissue, including muscle, and largely occurs due to a reprioritisation; lean mass is mobilised, releasing amino acids into circulation for preferential uptake by the liver to allow the synthesis of acute phase reactants, and the production of glucose from non-carbohydrate sources via gluconeogenesis. Hyperglycaemia is the result of peripheral and central insulin resistance. Peripheral insulin resistance refers to impaired insulin-mediated glucose uptake, whereas central insulin resistance refers to the inability of insulin to suppress glucose production from the liver [2].
Adequate pre-operative physiological reserve, commonly defined as the capacity for organs to function before exhaustion, is required to meet the functional demands of the surgical stress response, including increased cardiac output and delivery of oxygen [2, 3]. Likewise, pre-operative energy reserves, such as lean body mass, are required to support the stress-induced mobilisation of reserves so that physiological integrity and strength is not compromised [3, 4]. Surgical patients with low reserve, including malnourished, frail and sarcopaenic (muscle-depleted) patients, are vulnerable, with diminished capacity to respond to the added demands of a surgical insult [5, 6].
Malnutrition
There is no universally accepted definition for malnutrition; however, commonalities among definitions include an ‘unbalanced nutritional state’ that leads to ‘alterations in body composition’ and ‘diminished function’ [7]. An unbalanced nutritional state refers to both over- and undernutrition [8]. Patients who suffer from overnutrition consume excess energy, and patients who suffer from undernutrition consume too few nutrients, including energy and protein [8]. The ‘body composition’ term refers to anthropometric changes in total body and lean mass [1], whereas ‘function’, which most commonly refers to physical function, also encompasses cognitive and immune function [9].
In the Western world, undernutrition is seldom the
exclusive result of a deficient nutrient intake, and thus definitions for malnutrition often additionally include an aetiology-based diagnosis for malnutrition [9]. A definition of malnutrition for the undernourished surgical patient might thus be ‘a nutritional state in which nutrient intake does not match nutrient needs – due to underlying disease (s), the surgical stress response, chronic or acute inflammation, intestinal malabsorption (e.g. diarrhoea) and/ or patient-related factors (e.g. socio-economic status) – leading to losses in lean tissue and diminished function’.
Although it is important to be aware that malnutrition and undernutrition are not synonymous, the remainder of this article is restricted to malnutrition in the undernourished state.
Why avoid pre-operative malnutrition?
Nearly 50% of patients
admitted to hospital are malnourished or at risk of malnutrition [10]. If
malnutrition persists unabated, clinical problems ensue, including functional
impairment, decreased immune defence,
delayed wound healing and organ dysfunction [11]. Prospective cohort studies from around the world suggest that malnourished hospitalised and surgical patients have significantly worse clinical outcomes, including as much as fourfold greater risk of mortality [12–15]; greater odds of complications [12, 16–19]; more frequent re-admissions
[10, 12, 14, 20]; prolonged hospitalisations [10, 12, 14, 16, 19]; and increased healthcare costs [12, 21].
Experimental evidence indicates that malnutrition is a modifiable risk factor for surgery. A meta-analysis of 15 randomised controlled trials (RCTs), including 3831 malnourished patients undergoing a variety of surgical procedures, identified that peri-operative nutritional support was significantly more effective than the control at decreasing the incidence of infectious complications, with a risk ratio (RR; 95%CI) of 0.6 (0.5–0.7; p < 0.01); non- infectious complications 0.7 (0.6–0.9; p < 0.01); and shortening the length of hospital stay by approximately 2 days (95%CI -5.1 to -0.2; p < 0.05) [22]. A Cochrane review of 13 RCTs, including 548 patients, of pre-operative nutritional therapy in gastro-intestinal surgery found that pre-operative immune-enhancing nutrition compared with no or standard nutrition significantly reduced total postoperative complications, RR (95%CI) 0.7 (0.5–0.8;
p = 0.0006) [23]. The review also included 260
predominantly malnourished patients, in whom parenteral nutrition compared with no nutrition was also beneficial at reducing major complications, RR (95%CI) 0.6 (0.5–0.9; p = 0.005) [23]. Collectively, these studies indicate that both nutritional deficiencies and nutritional repletion have an impact on surgical recovery.
How does malnutrition develop?
Malnutrition is a nutritional state in which nutrient intake (from food, supplements, nutrition support) does not match nutrient needs, with multifactorial origins (Fig. 1) [8]. Impaired intake is considered the most important aetiological factor in the development of malnutrition [24], and can be its sole cause. Malnutrition may be related to disease and inflammatory processes altering nutrient requirements, rendering a previously adequate intake inadequate; disease- and treatment-related symptoms may also impede intake (referred to as nutrition-impact symptoms, for example, loss of appetite [25, 26]).
Before surgery, the onset of malnutrition might stem from a combination of the following: mechanical obstruction (e.g. tumour-related bowel obstruction); gastro- intestinal abnormalities (e.g. malabsorption); drug or treatment-related side-effects (e.g. nausea, intestinal failure from radiotherapy damage); metabolic abnormalities as a
result of primary and comorbid diseases (e.g. tumour- induced insulin resistance can mobilise endogenous energy sources such as amino acids); and several patient- related factors that have an impact on food intake (e.g. socio-economic status, social isolation, nutritional knowledge) [24, 25].
After
surgery, patients are also faced with several additional barriers to adequate
food intake, including the surgical
stress response and organisational barriers in
hospital (e.g. missed meals or tube feeds withheld due to scheduled clinical investigations). The
Canadian Malnutrition Task Force (CMTF), a prospective study involving 18 acute
care hospitals across
Canada, identified that nearly 50% of hospitalised patients
felt ‘too sick’ to eat, a
third of patients had difficulty opening food packages, two-thirds were not
given hospital food when meals were missed, and nearly half did not get help when needed
[26]. In fact,
even patients provided with standardised enhanced recovery after surgery (ERAS)
nutrition care did not meet minimally
adequate requirements for protein [27, 28],
and required nutritional
education to correct misconceptions that impeded adequate
nutrition in hospital [29]. The CMTF also
identified that most patients did not improve their
nutritional status during
hospitalisation, and that half of the patients who remained in hospital > 7 days were identified
as malnourished at discharge. Furthermore, 75% of malnourished patients did not receive
care from a dietician
during their hospital stay, and only 11% received dietetic care post-discharge [30–33].
What can be done to avoid malnutrition?
Nutritional management should begin pre-operatively to optimise nutritional status in preparation for the metabolic demands of surgical injury. Nutritional management should continue postoperatively to maintain nutritional status for supporting wound healing, improving the immune response and facilitate functional recovery [1, 2].
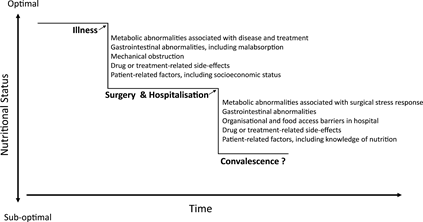
Figure 1 Diagram of potential deterioration in nutritional status over the peri-operative period. There are several peri-operative stages at which nutritional status could be compromised. The onset of disease and disease treatments may introduce metabolic abnormalities, including inflammation, that alter nutrition needs. Patients may find it difficult to meet their nutrient needs through food intake due to tumour-related obstruction, malabsorption and the onset of nutrition-impact symptoms (e.g. loss of appetite). Patient-related factors, including socio-economic status, additionally have an impact on food intake. Furthermore, malnutrition often goes undiagnosed, leaving the patient to face the surgical stress response in a suboptimal nutritional state, with diminished physiological reserves to respond to the demands of this stress response. In hospital, several barriers to adequate food intake exist, such as missed or interrupted meals, that have further impact on nutritional status. Patients are often discharged home without nutritional follow-up, they suffer further nutrition-impact symptoms from their pain medication and/or additional treatments, while relying on their own knowledge of food and nutrition to begin the process of convalescence
Surgical nutrition guidelines, such as the European Society for Clinical Nutrition and Metabolism [1], American Society of Parenteral Enteral Nutrition [34] and the American Society for Enhanced Recovery with Peri- operative Quality Initiative [35] all provide details on selecting nutrition screening tools, malnutrition assessment tools, and treatment for malnourished and at-risk patients. Nearly all guidelines suggest systematic, routine screening for malnutrition and subsequent nutrition assessment with a validated malnutrition assessment tool or a comprehensive nutrition assessment by a registered dietician if the nutrition screen is positive. A comprehensive nutrition assessment involves understanding the personal cause(s) of malnutrition and correcting barriers to adequate food intake. Patients identified as malnourished, or at risk, require individualised treatment plans that may include therapeutic diets (e.g. high protein), fortified foods, high protein oral nutrition supplements, enteral nutrition and/or parenteral nutrition [36].
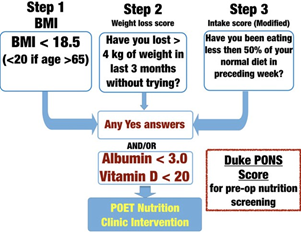
Figure 2 Duke University Pre-Operative Nutrition Score (PONS; adapted from reference [35]). PONS utilises data- driven questions from the validated Malnutrition Universal Screening Tool [37] to assess for malnutrition risk in peri- operative patients. Any score ≥ 1 signifies malnutrition risk, and the patient should receive pre-operative nutrition therapy before undergoing surgery
Examples of an existing tool, the Peri-operative Malnutrition Score [35, 37], used to identify malnutrition risk, and a nutrition optimisation programme for patients at risk of peri-operative malnutrition at Duke University, the Peri- Operative Enhancement Team clinic, are shown in Figs. 2 and 3.
Protein anabolism
Body proteins are constantly synthesised and degraded to maintain a neutral whole-body protein balance in normal, healthy adults [38]. The extent to which body proteins are broken down, releasing amino acids into circulation for reuse, is considerable; however, this recycling is not 100% efficient and, in particular, nine amino acids, referred to as essential or indispensable amino acids, cannot be synthesised de novo by adults, necessitating a daily requirement to ingest dietary protein [38]. When protein ingestion does not meet metabolic demands, catabolism (body protein breakdown) ensues to meet needs. When whole-body protein synthesis outweighs protein breakdown, anabolism is favoured [38].
Why support protein anabolism before surgery? Maintaining lean mass, including
muscle mass (the largest
‘reservoir’ of amino acids), is essential to support wound healing, immunity and autonomy [11, 39, 40]. Muscle-
depleted patients (i.e. sarcopaenic patients) have limited
reserve to respond to the surgical stress response [41, 42], increasing their odds of developing complications [6, 43], an increased length of hospital stay [43], and contributing to poor survival [6, 43, 44]. Computed tomography studies are beginning to define pre-operative body composition profiles, including low muscle mass, that predict surgical outcomes. Multivariable analysis of 805 colorectal cancer patients identified that low muscle mass before surgery was an independent predictor of overall survival; however, it was the presence of myosteatosis (fatty infiltration, an indicator of muscle quality), that was associated with prolonged hospital stay. The authors also identified that, in particular, obese patients with low muscle mass were more likely to suffer from 30-day morbidity and mortality rates [6]. These findings suggest that specific body composition profiles predict different surgical risks.
Experimental evidence supports the idea that prehabilitation, an anabolic intervention comprising exercise, nutrition, and psychological preparation in the waiting period before surgery, promotes a better surgical outcome [45, 46]. Much like training for a marathon, surgical prehabilitation employs multi-modal interventions in the pre-surgical period to fortify physiological reserve, and thus prepares patients emotionally and physically to withstand surgical insult [41]. Randomised controlled trials indicate multi-modal prehabilitation successfully improves a variety of surgical outcomes in abdominal surgery patients, including earlier return to baseline function [45–47]. A recent meta-analysis of nine prospective cohort and RCT studies of nutrition prehabilitation, with or without exercise, in colorectal surgery identified that receipt of any prehabilitation significantly reduced days spent in hospital compared with controls by 2 days (95%CI -3.5 to -0.9 days) [48]. Moreover, frail patients appear to gain the greatest benefits from prehabilitation treatment [45, 49]. As examples, colorectal cancer patients with poor baseline functional capacity experience more meaningful gains in pre- and postoperative function compared with patients with good baseline functional capacity [49]; and patients aged over 70 years with functional limitations (ASA physical status 3–4) suffer fewer postoperative complications after abdominal surgery if treated with personalised multi-modal prehabilitation, when compared with control patients [45].
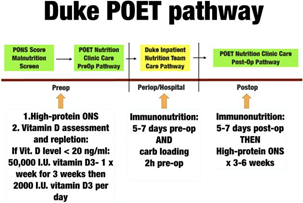
Figure 3 Pre-operative nutrition intervention plan from the Peri-operative Enhancement Team (POET) clinic at Duke University. PONS, Pre-Operative Nutrition Score. Any patient assessed as being at malnutrition risk (PONS score ≥ 1) is referred for evaluation by a dietitian and receives nutrition care according to this pathway. ONS, oral nutrition supplements.
How come surgical patients require pre-operative anabolic preparation?
Patients with illness, including surgical injury, inflammation and malignant disease, often present with an elevated turnover of body proteins, necessitating a greater total protein intake to attenuate the catabolism of body tissues to meet needs [2]. Additionally, disease- and treatment-related disruptions in normal metabolism have been found to alter specific amino acid requirements; suggesting that several amino acids, such as glutamine, arginine and cysteine, may become conditionally essential in oncological and surgical states [50, 51]. For instance, biosynthesis of acute phase proteins, associated with inflammation, imposes a new demand for aromatic and sulphur amino acids [50]. A stable isotope investigation estimated that, in pancreatic cancer, patients experiencing an ongoing inflammatory response,
2.6 g of muscle protein would need to be catabolised to synthesise 1 g of the positive acute phase reactant fibrinogen, if food was not consumed [51]. In addition, older patients [52] and patients with advanced cancer [53, 54] might suffer from anabolic resistance. That is, although their anabolic capacity is intact, a larger, sufficient dose of amino acids is required to achieve a typical anabolic response [52, 53]. If dietary intake does not compensate for these specific and total amino acid demands, body tissue is catabolised to meet needs (Fig. 4) [55].
Unfortunately, most older adults do not meet the minimal dietary protein requirements established for healthy individuals. A prospective cohort of 1793 community-dwelling older adults revealed that half the cohort consumed less than 1 g protein.kg-1day-1 [39]. Recent evaluations of dietary protein requirements propose that intakes in the range of at least 1.2–1.6 g kg-1day-1 are required to mitigate age-related muscle depletion and support optimal muscle health in ageing [39].
What can be done to meet protein needs and support protein anabolism before surgery?
Dietary protein
consumption and resistance exercise- training exert independent and additive
anabolic effects.
The hyperamino-acidaemia that follows protein ingestion without exercise stimulates a transient increase in muscle protein synthesis [56]; in healthy individuals, this anabolic effect is offset by daily catabolic periods (i.e. fasting between meals and during sleep) to produce an overall neutral protein balance that maintains lean mass [38]. Resistance exercise, even in the absence of food intake, stimulates muscle protein synthesis at 24 h and up to 48 h postexercise in certain populations [38, 57]. Resistance exercise, however, also elicits a concomitant increase in muscle protein breakdown for up to 24 h [57]. The net effect is that muscle protein balance after exercise improves, but does not become positive, in the fasted state (i.e. fasted state losses are less) [38]. Lean tissue accretion, however, will not occur without a positive protein balance, with protein synthesis exceeding protein breakdown. Stable isotope studies suggest net muscle protein balance postexercise remains negative until amino acids are available [38, 57, 58]. Instead, it is the synergistic effect of feeding- and exercise-induced stimulation of muscle protein synthesis that positively impacts protein balance, to a greater extent than either feeding or exercise could alone. Repeated bouts of resistance exercise and protein feeding stimulate lean tissue gains [38].
Several stable isotope studies show that the protein
synthetic response in muscle plateaus with the ingestion of a 20–35 g dose of protein – known as the ‘muscle full effect’ [59]. At this point, ingested amino acids are no longer used for muscle protein synthesis. This muscle full effect has been observed both after exercise [60, 61] and with meals at rest [62, 63]. Moore et al. used stable isotopes to measure muscle protein synthesis in six men who consumed 0 g, 5 g, 10 g, 20 g or 40 g whole egg protein on five separate occasions, so that each participant served as his own control, after an intense bout of leg-based resistance exercise. The authors found that muscle protein synthesis was maximally stimulated at 20 g [60].
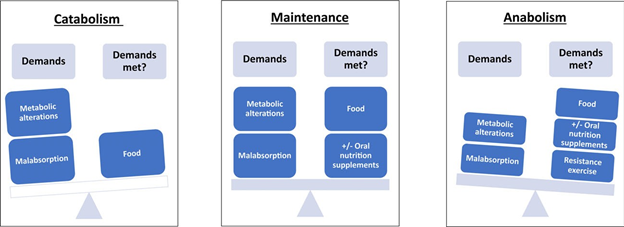
Figure 4 Diagram of the balance between peri-operative metabolic supply and demand. Patients with illness, including surgical injury, acute or chronic inflammation and cancer, often have greater needs for dietary protein. Elevated protein needs are the result of metabolic alterations associated with illness and illness-related treatments. When protein ingestion from food does not meet metabolic demands, body tissue is catabolised to meet needs. By meeting metabolic demands and maintaining homeostasis, largely through food intake, the patient avoids excessive catabolism and consequent losses of body protein and strength. Patients can achieve anabolism and build body tissue before surgery through adequate food/nutrient intake, adequate protein intake (from food and/or supplements), and resistance exercise.
Based on evidence of this ceiling effect in muscle, an ‘equal distribution hypothesis’ has been proposed [64]. This recommends that protein intake should be spread across all meals [63] and when eating after exercise [58], aiming for an amount of 20–35 g [59, 64]. It also suggests that the anabolic response is increased with habitual repetition. A 7-day crossover feeding study in healthy adult men by Mamerow et al. [65] supports this hypothesis. The authors found that 24 h mixed muscle protein synthesis was approximately 25% greater in response to an even distribution of protein (i.e. 30 g with meals) rather than a skewed protein distribution, despite diets being iso- energetic and isonitrogenous.
There
are some criticisms of the ‘equal distribution hypothesis’. An editorial stated
that it is premature to conclude
that an acute anabolic response accurately predicts the anabolic response over
the long-term [66]. Also, laboratory
settings do not reflect real life eating patterns; in reality, our meals are often composed
of mixed macronutrients rather than protein alone. The macronutrient composition of meal
intake may influence protein synthesis [59]. Finally, because the hypothesis is based
on studies of muscle protein
synthesis, which do not account for whole-body protein needs,
some authors
believe the total anabolic response may be underestimated [67].
Most often, ‘high-quality’ proteins are reported to exert the greatest effect on muscle protein synthesis [56]. According to a recent report by the Food and Agriculture Organization (FAO) of the United Nations, protein quality should be assessed based on the availability of essential amino acids after digestion in comparison with amino acid requirements [68]. The FAO has adopted the digestible indispensable amino acid score to quantify protein quality. Using this scoring system, animal proteins tend to constitute high-quality proteins, with milk proteins among the highest quality [68].
If we extrapolate all these findings from healthy populations, while recognising that they need to be confirmed in surgical settings, a protein-centred approach to meal planning that includes high-quality proteins and a relatively even daily distribution of protein intake might effectively maximise protein synthesis. This would require a change in eating habits; for instance, North Americans tend to consume > 60% of their daily protein at their evening meal [69], and thus would not achieve the ‘muscle full effect’ at their earlier meals. While the ‘equal distribution hypothesis’ is intriguing, whole-body protein needs should not be forgotten, and these might require > 35 g protein intake with meals. Optimal total daily protein intakes for surgery are currently not well defined, although several guidelines suggest that surgical patients should consume at least 1.2–2.0 g protein.kg-1.day-1 [1, 35].
Additional nutrients, such as omega-3 fatty acids and vitamin D, may also complement or augment the protein anabolic response. Smith et al. [70] randomly assigned 16 healthy, older adults to receive either omega-3 fatty acids or corn oil for 8 weeks. Corn oil supplementation had no effect on muscle protein synthesis rate, whereas omega-3 fatty acid supplementation was found to augment muscle protein synthesis. Likewise, a meta-analysis of 13 RCTs of supplemental vitamin D in adults aged > 60 years, compared with placebo or standard treatment on muscle function, found that supplementation with at least 800 IU of vitamin D decreased postural sway, reduced time to complete the Timed Up and Go Test, and marginally increased lower extremity strength [71]. These findings might also be applicable in clinical settings.
In conclusion, avoiding malnutrition and supporting anabolism are basic surgical nutritional goals. Before surgery, these goals can be met through nutrition screening and assessment to diagnose, treat and prevent malnutrition. Pre-operative nutritional interventions, such as nutritional prehabilitation with exercise cotherapy, function to optimise overall nutritional status and support protein anabolism before surgery, conditioning stronger patients for an earlier surgical recovery.
Acknowledgements
PW is an associate editor of Clinical Nutrition. PW has received grant funding related to this work from the NIH NHLBI R34 HL109369, Canadian Institutes of Health Research, Baxter, Fresenius, Lyric Pharmaceuticals, Isomark Inc., Medtronics and Cardinal. PW has served as a consultant to Abbott, Nestle Fresenius, Baxter, Medtronics, Cardinal, Nutricia, and Lyric Pharmaceuticals, and Takeda for research related to improving patient outcomes with nutrition. PW has limited ownership shares in Isomark for his consulting work with Isomark, which has otherwise been unpaid in nature. PW has received honoraria or travel expenses for lectures on improving nutrition care in illness from Abbott, Nutricia, Fresenius and Cardinal. CG – no competing interests declared.
References
- Weimann A, Braga M, Carli F, et al. ESPEN guideline: clinical nutrition in surgery. Clinical Nutrition 2017; 36: 623–50.
- Gillis C, Carli F. Promoting perioperative metabolic and nutritional care. Anesthesiology 2015; 123: 1455–72.
- Davies SJ, Wilson RJL. Preoperative optimization of the high- risk surgical patient. British Journal of Anaesthesia 2004; 93: 121–8.
- Fearon KC, Jenkins JT, Carli F, Lassen K. Patient optimization for gastrointestinal cancer surgery. British Journal of Surgery 2013; 100: 15–27.
- Amrock LG, Deiner S. Perioperative frailty. International Anesthesiology Clinics 2014; 52: 26–41.
- Malietzis G, Currie AC, Athanasiou T, et al. Influence of body composition profile on outcomes following colorectal cancer surgery. British Journal of Surgery 2016; 103: 572–80.
- Laur CV, McNicholl T, Valaitis R, Keller HH. Malnutrition or frailty? Overlap and evidence gaps in the diagnosis and treatment of frailty and malnutrition. Applied Physiology, Nutrition and Metabolism 2017; 42: 449–58.
- White JV, Guenter P, Jensen G, Malone A, Schofield M. Consensus statement of the Academy of Nutrition and Dietetics/American Society for Parenteral and Enteral Nutrition: characteristics recommended for the identification and documentation of adult malnutrition (undernutrition). Journal of the Academy of Nutrition and Dietetics 2012; 112: 730–8.
- Soeters P, Bozzetti F, Cynober L, Forbes A, Shenkin A, Sobotka
L. Defining malnutrition: a plea to rethink. Clinical Nutrition
2017; 36: 896–901.
- Jeejeebhoy KN, Keller H, Gramlich L, et al. Nutritional assessment: comparison of clinical assessment and objective variables for the prediction of length of hospital stay and readmission. American Journal of Clinical Nutrition 2015; 101: 956–65.
- Stratton RJ, Green CJ, Elia M. Disease-related malnutrition: an evidence-based approach to treatment. Wallingford, UK: CAB International Publishing, 2003.
- Tangvik RJ, Tell GS, Eisman JA, et al. The nutritional strategy: four questions predict morbidity, mortality and health care costs. Clinical Nutrition 2014; 33: 634–41.
- Guerra RS, Sousa AS, Fonseca I, et al. Comparative analysis of undernutrition screening and diagnostic tools as predictors of hospitalisation costs. Journal of Human Nutrition and Dietetics 2016; 29: 165–73.
- Agarwal E, Ferguson M, Banks M, et al. Malnutrition and poor food intake are associated with prolonged hospital stay, frequent readmissions, and greater in-hospital mortality: results from the Nutrition Care Day Survey 2010. Clinical Nutrition 2013; 32: 737–45.
- Vaid S, Bell T, Grim R, Ahuja V. Predicting risk of death in general surgery patients on the basis of preoperative variables using American College of Surgeons National Surgical Quality Improvement Program data. Permanente Journal 2012; 16: 10–17.
- Sorensen J, Kondrup J, Prokopowicz J, et al. EuroOOPS: an international, multicentre study to implement nutritional risk screening and evaluate clinical outcome. Clinical Nutrition 2008; 27: 340–9.
- Kwag S-J, Kim J-G, Kang W-K, Lee J-K, Oh S-T. The nutritional risk is a independent factor for postoperative morbidity in surgery for colorectal cancer. Annals of Surgical Treatment and Research 2014; 86: 206–11.
- Schiesser M, Kirchhoff P, Mu€ller MK, Scha€fer M, Clavien P-A.
The correlation of nutrition risk index, nutrition risk score, and bioimpedance analysis with postoperative complications in patients undergoing gastrointestinal surgery. Surgery 2009; 145: 519–26.
- Sun Z, Kong X-J, Jing X, Deng R-J, Tian Z-B. Nutritional risk screening 2002 as a predictor of postoperative outcomes in patients undergoing abdominal surgery: a systematic review and meta-analysis of prospective cohort studies. PLoS ONE 2015; 10: e0132857.
- Kassin MT, Owen RM, Perez SD, et al. Risk factors for 30-day hospital readmission among general surgery patients. Journal of the American College of Surgeons 2012; 215: 322–30.
- Curtis LJ, Bernier P, Jeejeebhoy K, et al. Costs of hospital malnutrition. Clinical Nutrition 2017; 36: 1391–6.
- Zhong J-X, Kang K, Shu X-L. Effect of nutritional support on clinical outcomes in perioperative malnourished patients: a
meta-analysis. Asia Pacific Journal of Clinical Nutrition 2015;
24: 367–78.
- Burden S, Todd C, Hill J, Lal S. Pre-operative nutrition support in patients undergoing gastrointestinal surgery. Cochrane Database of Systematic Reviews 2012; 11: Cd008879.
- Saunders J, Smith T, Stroud M. Malnutrition and undernutrition.
Medicine 2011; 39: 45–50.
- Nicolini A, Ferrari P, Masoni MC, et al. Malnutrition, anorexia and cachexia in cancer patients: a mini-review on pathogenesis and treatment. Biomedicine and Pharmacotherapy 2013; 67: 807–17.
- Keller H, Allard J, Vesnaver E, et al. Barriers to food intake in acute care hospitals: a report of the Canadian Malnutrition Task Force. Journal of Human Nutrition and Dietetics 2015; 28: 546– 57.
- Gillis C, Nguyen TH, Liberman AS, Carli F. Nutrition adequacy in enhanced recovery after surgery: a single academic center experience. Nutrition in Clinical Practice 2015; 30: 414–19.
- Yeung SE, Hilkewich L, Gillis C, Heine JA, Fenton TR. Protein intakes are associated with reduced length of stay: a comparison between Enhanced Recovery After Surgery (ERAS) and conventional care after elective colorectal surgery. American Journal of Clinical Nutrition 2017; 106: 44–51.
- Gillis C, Gill M, Gramlich L. Food is medicine: a qualitative analysis of patient barriers to food intake in an enhanced recovery after surgery (ERAS) setting. Applied Physiology, Nutrition, and Metabolism 2018; 43: S13.
- Allard JP, Keller H, Jeejeebhoy KN, et al. Decline in nutritional status is associated with prolonged length of stay in hospitalized patients admitted for 7 days or more: a prospective cohort study. Clinical Nutrition 2016; 35: 144–52.
- Allard JP, Keller H, Teterina A, et al. Factors associated with nutritional decline in hospitalised medical and surgical patients admitted for 7 days or more: a prospective cohort study. British Journal of Nutrition 2015; 114: 1612–22.
- Keller H, Allard JP, Laporte M, et al. Predictors of dietitian consult on medical and surgical wards. Clinical Nutrition 2015; 34: 1141–5.
- Keller H, Payette H, Laporte M, et al. Patient-reported dietetic care post hospital for free-living patients: a Canadian Malnutrition Task Force Study. Journal of Human Nutrition and Dietetics 2018; 31: 33–40.
- Mueller CM, Lord LM, Marian M, McClave S. The ASPEN adult nutrition support core curriculum. Silver Spring: American Society for Parenteral and Enteral Nutrition, 2017.
- Wischmeyer PE, Carli F, Evans DC, et al. American society for enhanced recovery and perioperative quality initiative joint consensus statement on nutrition screening and therapy within a surgical enhanced recovery pathway. Anesthesia and Analgesia 2018; 126: 1883–95.
- Keller HH, McCullough J, Davidson B, et al. The integrated nutrition pathway for acute care (INPAC): building consensus with a modified Delphi. Nutrition Journal 2015; 14: 63.
- BAPEN. The ‘MUST’ report, 2003. www.bapen.org.uk/scree ning-and-must/must/must-report (accessed 09/08/2018).
- Phillips SM. Protein requirements and supplementation in strength sports. Nutrition 2004; 20: 689–95.
- Phillips SM, Chevalier S, Leidy HJ. Protein “requirements” beyond the RDA: implications for optimizing health. Applied Physiology, Nutrition and Metabolism 2016; 41: 565–72.
- Malietzis G, Johns N, Al-Hassi HO, et al. Low muscularity and myosteatosis is related to the host systemic inflammatory response in patients undergoing surgery for colorectal cancer. Annals of Surgery 2016; 263: 320–5.
- Carli F, Gillis C, Scheede-Bergdahl C. Promoting a culture of prehabilitation for the surgical cancer patient. Acta Oncologica 2017; 56: 128–33.
- Dello SAWG, Lodewick TM, van Dam RM, et al. Sarcopenia negatively affects preoperative total functional liver volume in patients undergoing liver resection. Hepato-pancreatic-biliary Journal 2013; 15: 165–9.
- Kazemi-Bajestani SMR, Mazurak VC, Baracos V. Computed tomography-defined muscle and fat wasting are associated with cancer clinical outcomes. Seminars in Cell and Developmental Biology 2016; 54: 2–10.
- Martin L, Birdsell L, Macdonald N, et al. Cancer cachexia in the age of obesity: skeletal muscle depletion is a powerful prognostic factor, independent of body mass index. Journal of Clinical Oncology 2013; 31: 1539–47.
- Barberan-Garcia A, Ubre M, Roca J, et al. Personalised prehabilitation in high-risk patients undergoing elective major abdominal surgery: a randomized blinded controlled trial. Annals of Surgery 2018; 267: 50–6.
- Gillis C, Li C, Lee L, et al. Prehabilitation versus rehabilitation. A randomized control trial in patients undergoing colorectal resection for cancer. Anesthesiology 2014; 121: 937–47.
- Bousquet-Dion G, Awasthi R, Loiselle S-E’, et al. Evaluation of
supervised multimodal prehabilitation programme in cancer patients undergoing colorectal resection: a randomized control trial. Acta Oncologica 2018; 57: 849–59.
- Gillis C, Buhler K, Bresee L, et al. Effects of nutritional prehabilitation, with and without exercise, on outcomes of patients who undergo colorectal surgery: a systematic review and meta-analysis. Gastroenterology 2018; 155: e4.
- Minnella EM, Awasthi R, Gillis C, et al. Patients with poor baseline walking capacity are most likely to improve their functional status with multimodal prehabilitation. Surgery 2016; 160: 1070–9.
- Cynober LA. Metabolic & therapeutic aspects of amino acids in clinical nutrition, 2nd edn. Boca Raton, FL: CRC Press, 2004.
- Preston T, Slater C, McMillan DC, Falconer JS, Shenkin A, Fearon KCH. Fibrinogen synthesis is elevated in fasting cancer patients with an acute phase response. Journal of Nutrition 1998; 128: 1355–60.
- Moore DR, Churchward-Venne TA, Witard O, et al. Protein ingestion to stimulate myofibrillar protein synthesis requires greater relative protein intakes in healthy older versus younger men. Journals of Gerontology Series A 2015; 70: 57–62.
- Winter A, MacAdams J, Chevalier S. Normal protein anabolic response to hyperaminoacidemia in insulin-resistant patients with lung cancer cachexia. Clinical Nutrition 2012; 31: 765–73.
- Engelen MPJ, van der Meij BS, Deutz NEP. Protein anabolic resistance in cancer: does it really exist? Current Opinion in Clinical Nutrition and Metabolic Care 2016; 19: 39–47.
- Williams JP, Phillips BE, Smith K, et al. Effect of tumor burden and subsequent surgical resection on skeletal muscle mass and protein turnover in colorectal cancer patients. American Journal of Clinical Nutrition 2012; 96: 1064–70.
- Tang JE, Phillips SM. Maximizing muscle protein anabolism: the role of protein quality. Current Opinion in Clinical Nutrition and Metabolic Care 2009; 12: 66–71.
- Phillips SM, Tipton KD, Aarsland A, Wolf SE, Wolfe RR. Mixed muscle protein synthesis and breakdown after resistance exercise in humans. American Journal of Physiology 1997; 273: E99–E107.
- Biolo G, Tipton KD, Klein S, Wolfe RR. An abundant supply of amino acids enhances the metabolic effect of exercise on muscle protein. American Journal of Physiology 1997; 273: E122–E129.
- Morton RW, McGlory C, Phillips SM. Nutritional interventions to augment resistance training-induced skeletal muscle hypertrophy. Frontiers in Physiology 2015; 6: 245.
- Moore DR, Robinson MJ, Fry JL, et al. Ingested protein dose response of muscle and albumin protein synthesis after
resistance exercise in young men. American Journal of Clinical Nutrition 2009; 89: 161–8.
- Witard OC, Jackman SR, Breen L, Smith K, Selby A, Tipton KD. Myofibrillar muscle protein synthesis rates subsequent to a meal in response to increasing doses of whey protein at rest and after resistance exercise. American Journal of Clinical Nutrition 2014; 99: 86–95.
- Symons TB, Sheffield-Moore M, Wolfe RR, Paddon-Jones D. A moderate serving of high-quality protein maximally stimulates skeletal muscle protein synthesis in young and elderly subjects. Journal of the American Dietetic Association 2009; 109: 1582–6.
- Areta JL, Burke LM, Ross ML, et al. Timing and distribution of protein ingestion during prolonged recovery from resistance exercise alters myofibrillar protein synthesis. Journal of Physiology 2013; 591: 2319–31.
- Paddon-Jones D, Campbell WW, Jacques PF, et al. Protein and healthy aging. American Journal of Clinical Nutrition 2015; 101: 1339S–1345S.
- Mamerow MM, Mettler JA, English KL, et al. Dietary protein distribution positively influences 24-h muscle protein synthesis in healthy adults. Journal of Nutrition 2014; 144: 876–80.
- Rodriguez NR. Protein-centric meals for optimal protein utilization: can it be that simple? Journal of Nutrition 2014; 144: 797–8.
- Kim I-Y, Schutzler S, Schrader A, et al. Quantity of dietary protein intake, but not pattern of intake, affects net protein balance primarily through differences in protein synthesis in older adults. American Journal of Physiology. Endocrinology and Metabolism 2015; 308: E21–E28.
- Wolfe RR. Update on protein intake: importance of milk proteins for health status of the elderly. Nutrition Reviews 2015; 73(Suppl 1): 41–7.
- Arentson-Lantz E, Clairmont S, Paddon-Jones D, Tremblay A, Elango R. Protein: a nutrient in focus. Applied Physiology, Nutrition and Metabolism 2015; 40: 755–61.
- Smith GI, Atherton P, Reeds DN, et al. Dietary omega-3 fatty acid supplementation increases the rate of muscle protein synthesis in older adults: a randomized controlled trial. American Journal of Clinical Nutrition 2011; 93: 402–12.
- Muir SW, Montero-Odasso M. Effect of vitamin D supplementation on muscle strength, gait and balance in older adults: a systematic review and meta-analysis. Journal of the American Geriatrics Society 2011; 59: 2291–300.